This project is subdivided into 7 work packages:
Work Package 1: Carbon Capture and a decision support tool
There are many CO2 capture technologies including post-combustion, pre-combustion, oxy-fuel combustion as well as Direct Air Capture (DAC). Deciding which technology to use might not be an easy task. The potential of the technologies will therefore be assessed through techno-economic analysis, life cycle assessment as well as estimation of potential social impact. There are three criteria to evaluate each technology which are engineering, economics, and environmental criteria. Within each criterion, there are Key Performance Indicators (KPIs) such as Technology Readiness Levels (TRLs), CO2 avoidance cost, footprints, etc. The different technologies will be ranked based on their performances under the criteria and KPIs. This will provide an easy-to-read Decision Support Tool (DST) to identify the most relevant CO2 capture applications. The study will focus on Belgian emissions and will discuss specific case studies, supplying material for the other project partners targeting CO2 re-use.
Work Package 2: Power to chemicals
In PROCURA, a comparison of the production modes of important (bulk) chemicals that can be produced out of CO2 or with a low carbon footprint, is made. This will be based on the unavoidable production costs, such as minimal required CO2 feedstock and energy cost and involve chemicals, obtained via low or high energy-demanding pathways and selected from a market perspective and CO2 mitigation potential.
For each chemical, the process technologies, at different Technology Readiness Levels, are summarized and feasible production routes are identified.
The potential of CO2-to-chemicals value chains for given energy scenarios will be evaluated by:
- Taking into account local conditions with regard to the renewable energy transition (energy storage and dedicated production);
- Mapping out stakeholders (CO2 emitters, energy suppliers, chemical producers, end-users, logistics and transport);
- Performing a generalized techno-economic assessment that includes the unavoidable process costs, efficiency of energy and feedstock use, next to fixed capital costs.
- Including an evaluation of technical, economic, policy and market-related hurdles towards its deployment.
A specific example:
- In the first period, the Power-to-Methanol scenario of Port of Antwerp was assessed and validated with regards to the value chain’s techno-economic feasibility.
- The extension of the ‘local’ Power-to-Methanol analysis towards scenarios that involve the import of renewable hydrogen or methanol, valorization in Belgium and comparison with the benchmark scenario is envisaged in the further steps;
- The environmental impact of the different scenarios will also be assessed, according to the ISO standard European LCA methodology, and will finally be accompanied by a proper supply chain analysis with VITO’s MooV tool.
- Ammonia was selected as the second molecule, based on the attention it deserved in Power-to-X roadmap studies and context analyses. The first techno-economic analysis was performed and compared with the methanol base case scenario.
Work Package 3: Power to energy
The main goal of work package 3 is to model the effects of deep decarbonisation of the Belgian energy system, using the IEA-ETSAP TIMES techno-economic equilibrium energy system model. Besides using literature sources, this work package will benefit from the output of the other six work packages. So far, we have set up the first version of a simple model, based on the existing so-called TIMES-BE energy system. Besides electrification of processes, we also look into green molecules such as hydrogen, methanol, ammonia and others. These molecules can either be imported e.g.: via the Port of Antwerp or produced onsite and used across a wide spectrum of industrial applications. But also other sectors such as transport, residential and commercial heating and power generation are investigated to see if they could benefit from molecules to further decrease the carbon footprint of the overall system at an optimal economical cost. In parallel, the so-called blue routes, carbon capture and storage as well as carbon capture and usage, are added to the model. Elements of circularity and also avoidance of locked in scenarios are some of the opportunities we are looking for. Right now, a number of storylines are being developed in order to run different scenarios at various time horizons. As the project develops and delivers more insights and results, the model will be enriched with both data and complexity in order to provide a number of options for policymakers, industry and other interested partners as a means to look into the future (2050 – 2060), in line with European and national projected climate goals.
Work Package 4: Solar fuels
Work package 4 performs a technology screening of solar fuel concepts.
Solar fuel technologies directly convert sunlight into hydrogen or other molecules. They are distinct from Power-to-X technologies, which require (grid) electricity, and from biofuels, which require biomass. Solar fuel technologies come in many different shapes and flavours. Some absorb solar energy to drive chemical reactions at very high temperatures while others convert solar energy into electrical charges, much like a solar cell. In photobiological approaches, bacteria are used to directly produce chemicals. Solar fuel technologies can be small scale or large scale. Many approaches are still at an early stage, while some are already quite mature.
Work package 4 will assess the status of existing solar fuel technologies and forecast their potential. This will allow a reasonable integration of promising technologies in energy roadmaps. Solar fuel technologies are dedicated technologies for renewable fuel production and are not directly linked to the electricity market. Due to their specificity, additional input to the other WPs will be required for proper model integration. This interaction will run throughout the project. Because of the fast development cycle of these technologies, the state of the art will be closely monitored during the whole project.
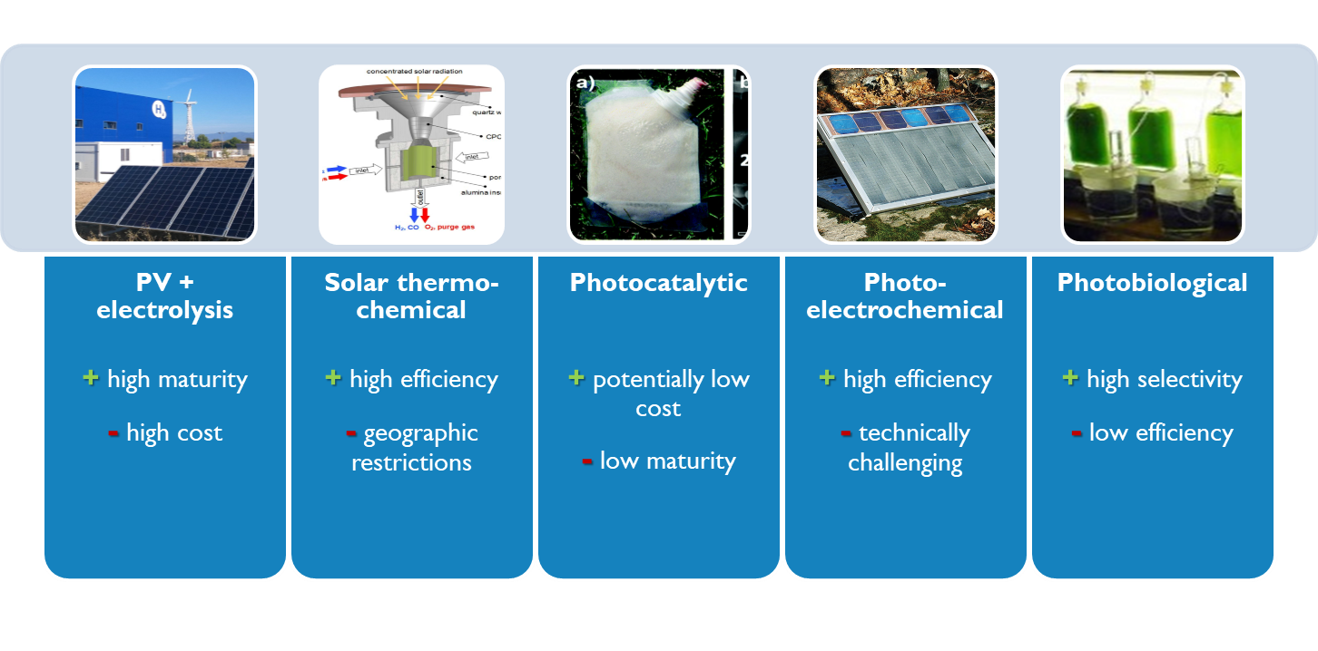
Perovskite solar cells (PSCs)
Perovskite solar cells (PSCs) are a very young PV technology which in only a few years has achieved impressive PCE values surpassing most established technologies. However, it has not found a way into industry because cost-effective upscaling and long-term stability have not yet been proven. Nonetheless, PSCs still draw great interest as an emerging thin-film PV technology mainly due to its simple deposition methods and potential for applications which require low weight and flexibility.
PSCs also offer a plausible way to overcome the Shockley-Queisser efficiency limit for single-junction solar cells. Absorption and thermalisation losses set a limit to the maximum attainable efficiency of single-junction devices. When stacking two bandgap-matched absorbers in what is called a “tandem” solar cell, these losses are significantly reduced. Integration of a PSC on top of a c-Si cell could allow commercial PV with higher efficiencies in a cost-effective manner, as long as the gain in PCE wins over the additional manufacturing costs. This material combination is attractive because it leverages the market dominance of silicon and utilizes the rapidly developing perovskite technology, which is highly bandgap-tuneable and uses cheap and abundant materials.
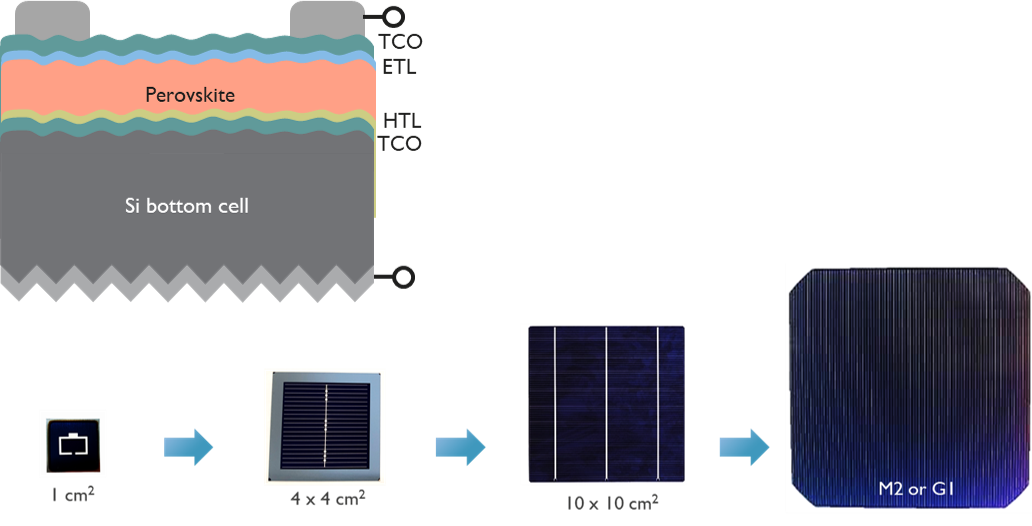
Work Package 5: Demonstrator H2O/CO2 reduction
Work package 5 deals with the design and construction of a demonstrator plant that is capable of converting CO2 into valuable chemicals. Direct electrochemical reduction of CO2 offers a great way to shave off power peaks in electricity grids dominated by renewable energy such as wind and solar. Electricity is used directly to reduce CO2 at the cathode. The anodic reaction is in most cases the oxidation of water to oxygen. Formic acid and methanol were the designated chemical for electrochemical reduction. Typically, formic acid forms preferably in aqueous electrolytes (i.e. dissolved CO2), methanol rather than in gaseous systems (i.e. humidified CO2).
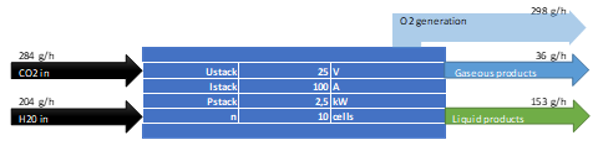
Based on the simplified process flow diagram a more detailed process scheme of the balance of plant (BoP) was drawn. From this detailed P&ID a complete list of components is created and this marks the start of procurement and finally the assembly of the full pilot plant. To date (September 2021) a high-cube 20ft container is prepared with a customized rack to install all the components, such as tanks, condensors, heating, cooling, piping, instrumentation, etc. During the coming weeks and months the construction will be finalized and the pilot system will be tested on-site for functional control and checking all safety features are in place. Once this is completed, the pilot can be deployed on any industrial site where a CO2 source and electricity is available. The future looks green.
Work Package 6: Comparative analysis for other countries
The aim of this work package is to analyse the possible strategies for Belgium regarding power-to-x, i.e. domestic production of hydrogen and derived products compared to the import of hydrogen carriers from countries with more renewable energy sources.
For this analysis, the hydrogen strategies of our neighbouring countries have been analysed, which shows that e.g. in Germany and the Netherlands both domestic production (opportunities for own companies to develop the technology) and import (opportunities for the seaports, ambition to become an H2 hub for the EU) are put forward. France is focusing on its own production and Spain is planning to become a net exporter of hydrogen.
To develop possible scenarios for Belgium, formal collaboration with the Hydrogen Import coalition (PoA, Deme, Engie, Fluys, MBZ, Exmar and WaterstofNet) has been set up. The coalition has analysed the entire import and transport chain of hydrogen, from various locations such as Chile, Oman, Australia and Morocco and calculated the Levelized cost of hydrogen for 2035 and 2050. Within the Procura project (link with work package 3), these projected costs of imported hydrogen are compared to domestic hydrogen production scenarios and alternative pathways (e.g. using methane with CCS, electrification…) in different use cases (e.g ammonia and methanol production, steel production etc…). Also, the influence of policy instruments (e.g. ETS or other support mechanisms) on the import vs own production scenarios will be modelled.
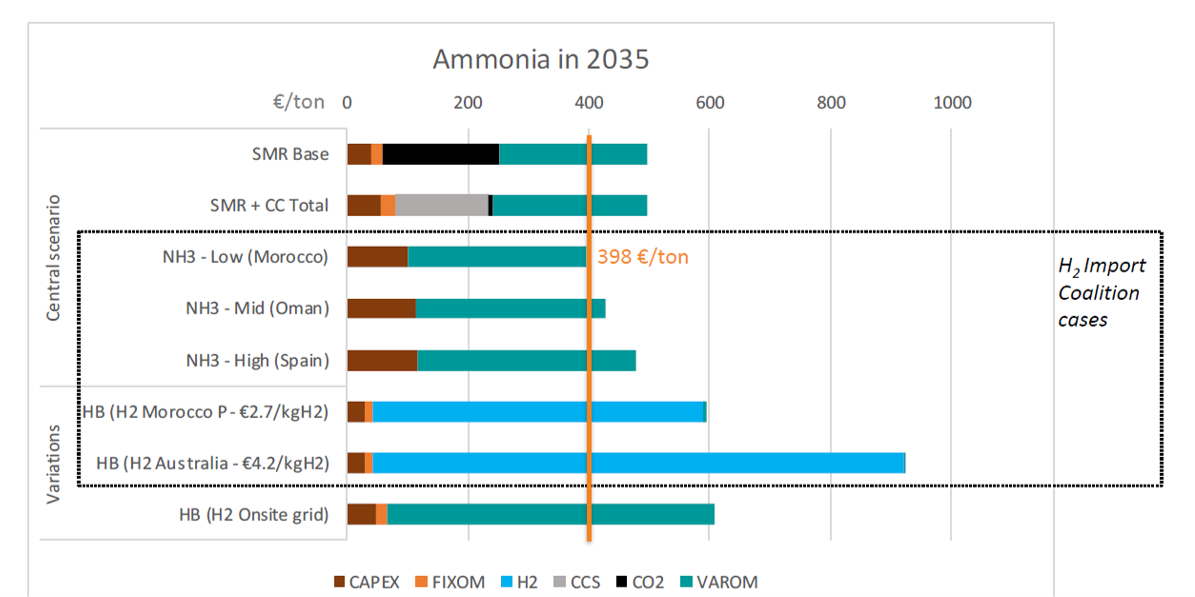
Work Package 7: Valorisation potential, market analysis and enabling conditions
Work package 7 looks at the valorisation potential, market analysis and enabling conditions for power to x, carbon capture and utilization or storage and other industrial mitigation technologies towards achieving climate neutrality.
The work package consists of three parts:
- WP 7.1: Identify synergies and symbiosis between the energy sector and industrial sectors
- WP 7.2 Identify (economic and other) opportunities and valorisation in the technological shift to H2, CCU and circular carbon from a value chain perspective
- WP 7.3 Identify the enabling conditions for the industrial transition towards H2, CCU, circular carbon
Specifically, the WP will identify examples of possible improved power and heat symbiosis and smart integration of industrial processes to minimise the loss of carbon to the atmosphere. Secondly, the WP will show through important value chain examples how much the final consumer cost impact will be if the production of basic materials (e.g. steel, plastics, fertilisers) shifts to climate neutrality and how policy can enable this transition. Finally, the WP will look at the results of other parts of the PROCURA research and see how infrastructure needs for Belgium could change towards achieving climate neutrality by 2050.